This text can be summarized in three points.
Nord Stream, if fixed, would supply as much energy as 73 nuclear reactors.
The initial thrust from exiting gas after the explosions exceeded that of a Falcon space rocket.
In the year since the explosions, human flatulence has emitted the same amount of methane to the atmosphere as was released from the three ruptured pipelines.
To get a feel for the magnitude of something, you need to compare it to something of similar magnitude which you know. I miss this type of elaboration in the reporting around the Nord Stream sabotage, and I’ve noticed that most people have no idea about the basic magnitudes important to the event. Therefore I’m writing this post although most of it is probably trivial and boring to many readers.
It’s often reported that the Nord Stream 1&2 pipelines were designed to deliver 110 “bcm” of natural gas annually. A bcm means a billion cubic meters of gas, but how much is that really? How are we supposed to put this number in a context where we can make relevant comparisons? I don’t care about volume, I want to know how much energy it is so I can compare it to the production by other means and the consumption of different countries.
Looking up bcm in Wikipedia I’m relieved to see “bcm” is not a measure of volume but one of energy. One bcm of Russian natural gas is 10.6 TWh of energy. So there it is, the Nord Stream pipelines would have delivered 1166 TWh of energy per year. TWh is the standard unit for reporting energy use on the country level, so all of a sudden we can make a lot of comparisons and get a good feel for how much energy supply was actually lost by the Nord Stream sabotage.
The 1166 TWh is the heat content of Nord Stream gas delivered in a year at full capacity, and if all of it was used for electricity production in modern German power plants it would render 641 TWh of electricity annually. That’s more than Germany's total electricity consumption of 500 TWh. Wind and solar in Germany is about 170 TWh, which is impressive, but it isn’t making up for the loss of 640 TWh, and gas has a lot of other good qualities too. It burns clean and can be used for direct heating with small investment. The gas power plants can be dispatched quickly to balance the unpredictable wind and solar, and the waste heat of the power plants can be recovered for residential heating since, unlike a nuclear plant, a gas plant can be placed where the heat is needed. No matter how you count, the loss was huge.
Nord Stream 1&2 could have delivered a third of Germany’s total energy needs and all of its electricity. One of the four Nord Stream pipes would be enough to replace all of Germany’s wind and solar power. To replace Nord Stream’s ability to produce 640TWh electric energy with nuclear reactors you would need 73 of them. (one GW is a typical electric power output of a nuclear reactor which corresponds to 8.76 TWh if it runs continuously the full year). Germany has about 30000 wind turbines producing 123 TWh annually. To match the annual energy production of the Nord Stream pipelines, Germany would need 156000 wind turbines (assuming they are of the same size as the current ones, and ignoring that fact it doesn’t work to have such a big share from intermittent sources).
Apparently, five men and a woman on a sailboat were able to terminate the equivalent of 73 nuclear power plants or 165000 wind turbines with a few kilos of explosives. It was a big bang for the buck, so I guess we shouldn’t be surprised that “intelligence operatives” think this was "a neat operation" .
The Power of the Compressed Gas
It takes energy to transport energy and gas pipelines are no exception. To transport the equivalent power of 73 nuclear reactors, you need the equivalent of at least one nuclear reactor to compress the gas and push it through the pipelines. In absolute terms, 1 GW of net power output just to compress gas is a lot and it should give us a hint that a lot of power was unleashed when the pipelines ruptured and the gas suddenly expanded.
At 19:04 on September 26, the Chief Officer of Cellus, a German cargo ship, saw a gigantic plume rise up from behind the horizon. He called his captain, Helge Jürgensen who took a photo with his iPhone 8 minutes later. They were then 6.9 nautical miles away, and the water fountain had lowered and widened. Captain Jürgensen sent me the original photo with metadata . He pinched his fingers on the iPhone screen to zoom to 3.8x before taking the photo. That’s “35mm sensor 100mm equivalent focal length”, allowing us to compute each pixel to be 1.1x1.1 meters and the water fountain could be determined to be 350 meters wide and 56 meters high.
Helge Jürgensen mapped out the positions of the plumes, warned other ships and contacted the authorities with a finished analysis that both plumes were right above the Nord Stream 1 pipeline. That’s how the Swedish JRCC got to know about the explosions so they could issue a marine warning and inform other agencies. When Captain Jürgensen took the photo above, the rarefaction wave traveling at 400 meters per second inside the pipeline, had not yet reached the German shore. The pipeline operator had no means of knowing anything was wrong. The military with its network of hydrophones would know pretty soon as the sound travels almost 4 times as fast in the water, but all we know for sure is that they didn’t bother to tell anyone.
I’m getting back to the power of the blast in the last section of this text with a quick update on our effort to simulate the fluid dynamics of the event. But first a note on the environmental impact.
The Methane Release
518000 tons of natural gas was released from the three ruptured pipelines and almost exactly 500000 tons of that gas was methane. It’s almost trivial to compute this number, but just almost, not quite. There’s a little caveat to computing the density of natural gas which I’d like to point out before trying to put the 500000 tons in perspective.
The geometry of the pipelines is readily available on the web. NS1 were two 1224 km long cylinders with a cross-sectional area of 1.044 square meters. NS2 was one cylinder 1230 km long. The Swedish coast guard gave me this spreadsheet which shows the starting and final pressures of each pipeline. The surprise (for me), was that natural gas doesn’t behave like an “ideal gas” at all. The product of pressure and volume isn’t constant. One cubic meter of natural gas compressed to 165 atmospheres doesn’t have the same mass as 165 cubic meters of gas at 1 atmosphere. At 165 bars and 6 degrees Celsius (average bottom temperature along the pipes) you get 35% more gas into the pipeline than you would if the gas was “ideal”. You need to compress 223.5 cubic meters of natural gas from 1 bar to 165 bar for the volume volume to be 1 cubic meter. Big difference. Luckily there are online calculators allowing you to compute the density and get the correct answer.
So now, how how much is half a million tonnes of methane?
In terms of volume it is 0.73 billion cubic meters which isn’t much compared to the 110 billion cubic meters which the Nord Stream pipelines could deliver per year. You can argue that losing 0.66 percent of your annual gas production in a worst case assault is an acceptable risk. The environmental impact must always be put in relation to the utility, and just looking at the absolute amount isn’t a good metric.
But methane is known to be a nasty greenhouse gas trapping 84 times more heat than CO2 for the same mass, so how does the Nord Stream emissions compare to other sources of atmospheric methane? I found this blogpost to answer this and many other questions relating to atmospheric methane. The post reveals that the world’s livestock emit 120 million tonnes of methane every year, so not even during the 3 days of heavy outgassing in the Baltic was Nord Stream able to outpace our burping cows. Even human flatulence is just about to match the Nord Stream emissions. The blogpost teaches us that since the attack a year ago, the human race has farted half a million tons of methane -exactly the amount of emissions from the Nord Stream ruptures.
Simulating the Event
We’re far from being able to simulate the full mechanics of the event, but I think it’s possible and worth pursuing. A full simulation would have to involve not just the gas flow and thrust, but the structural mechanics of the pipeline and the fluid dynamics of the surrounding water. The shape of the seafloor and spread of debris bear a many clues and could verify if we get all these parts of the physics right. I’m intrigued by the fact that the NS1A and NS1B pipelines, both pressurized to 165 bars, have left almost identical marks on the seafloor. NS2AD is much smaller and NS2AS smaller still. It seems like the effect is almost entirely a function of pressure and the size of the bombs didn’t matter. I also believe that just one bomb was placed near the middle of each site, but it would be good to know for sure, so we keep working on it.
The first step is to simulate the gas flow out of the pipeline. My son Aron was kind enough to help his dad with a Matlab program that does just that. You can check it out yourselves here: https://github.com/arona888/Shock-Tube.git
The simulation reveals that when the Nord Stream 1 pipeline was split up, gas was erupting at the speed of sound with a mass flow rate of 19.5 tons per second from each end. The first second after the split, 39 tons of gas was coming out with a kinetic energy of 3 billion watt-seconds, so the “power” of the flow was 3GW, or the equivalent of the power output from three nuclear power plants. That’s a lot of power and it’s not even counting the power from the subsequent expansion of the gas which was still at 50 atmospheres when leaving the pipe.
The graph above shows the mass flow out of one pipe end of NS1 which was pressurized to 165 atmospheres.
19.5 tons per second at sonic speed is a quite impressive number if you’re into jet propulsion. The mass flow times the change of speed equals thrust and in this case it would be 19500 (kg/s) *400 (m/s) = 7800000 N. That’s about 800 metric tons, and on top of that the cold gas erupting at the speed of sound still has a pressure of 50 bars adding another 500 metric tons to the thrust from a 1 square meter opening. The total thrust of 1300 metric tons is roughly twice the thrust from a SpaceX Falcon 9 space rocket.
No wonder the pipeline was torn in pieces and thrown around. The seafloor has traces of a breakup sequence starting with an explosion at the center of each site. My guess is that this initial phase was settled in the first minute leading to two open ends 250 meters apart on NS1 and about 45 meters apart for NS2AD .
When Helge Jürgensen took the picture 8 minutes after the blast, the total flow from the two ends had already sunk to 8 ton/s from the initial 39 ton/s . When the first aerial footage was taken 4 hours later from a Danish military helicopter dispatched from Roskilde airport, the flow was down to 400 kg/sec from the German end and 1200 kg/s from the Russian end.
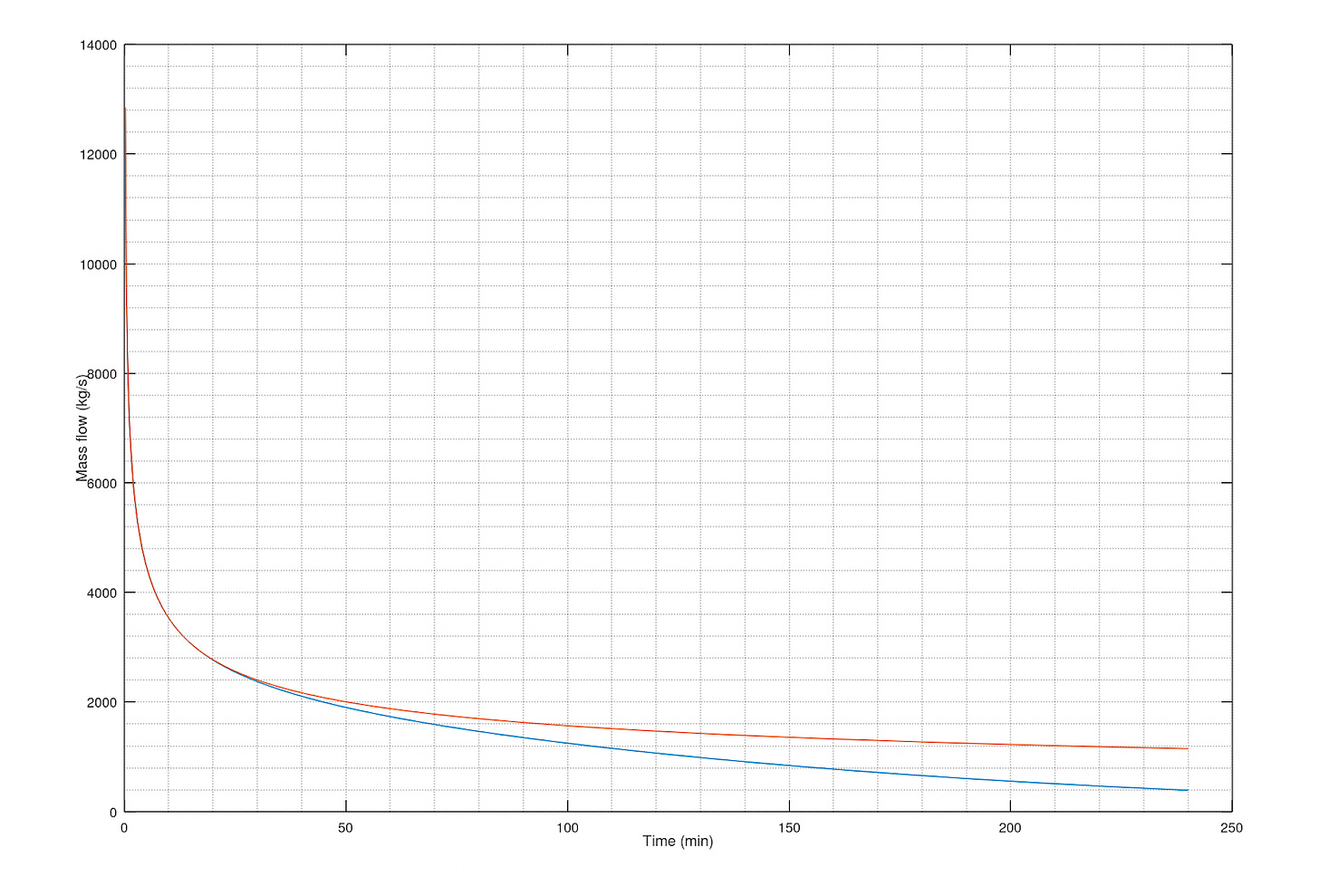
In the graph above, the brown curve represents the gas flow out of the Russian end and the blue is the flow out of the pipe section coming from Germany. The sound has to travel 230km to Germany and back again before the flows from the two ends can start to separate.
Bubble Plumes
The giant bubble plumes above the leaks are fascinating in their own right. I couldn’t find any relevant research on the subject but Göran Broström at the marine science department of Gothenburg University sent me a quite relevant paper with the title Very Large Deep-Set Bubble Plumes From Broken Gas Pipelines . Fanneløp and Bettelini had tried back in 2007 to figure out what would happen if a pressurized gas pipeline ruptured completely under water. I don’t know if the authors are still alive or if their calculations were preserved anywhere in the memory of pipeline operators. The risk of a complete rupture seems to have been considered too small to be listed as a scenario in Nord Stream emergency procedures. That’s what I’ve heard anyway.
Small bubble plumes are well known and used as water “gravity pumps” by divers and offshore workers. When gas bubbles are immersed in the water, the resulting mix is lighter than the surrounding water so it becomes buoyant and rises in a “plume”, just like a plume of hot air rises in a chimney and continues straight up in the air when there is no wind. To send water 56 meters up in the sky, it needs to have a speed of 33 meters per second at the surface. That’s 120 km per hour, faster than you’re allowed to drive on the motorways in Sweden. That speed is quite consistent with Fanneløp and Bettelinis calculations although they don’t consider any scenario with such huge mass flows as Nord Stream had the first few minutes. When the photos and films were taken 4 and 17 hours later the 400kg/s flow led to 20 meter high fountains. Fanneløp concludes that the plume is unstable, sometimes narrow and sometimes wider and this causes the geyser type eruptions. It’s also often called a “boil”. Fanneløp wanted to provide a tool to estimate the mass flow from the pipe based on the height of the plume.
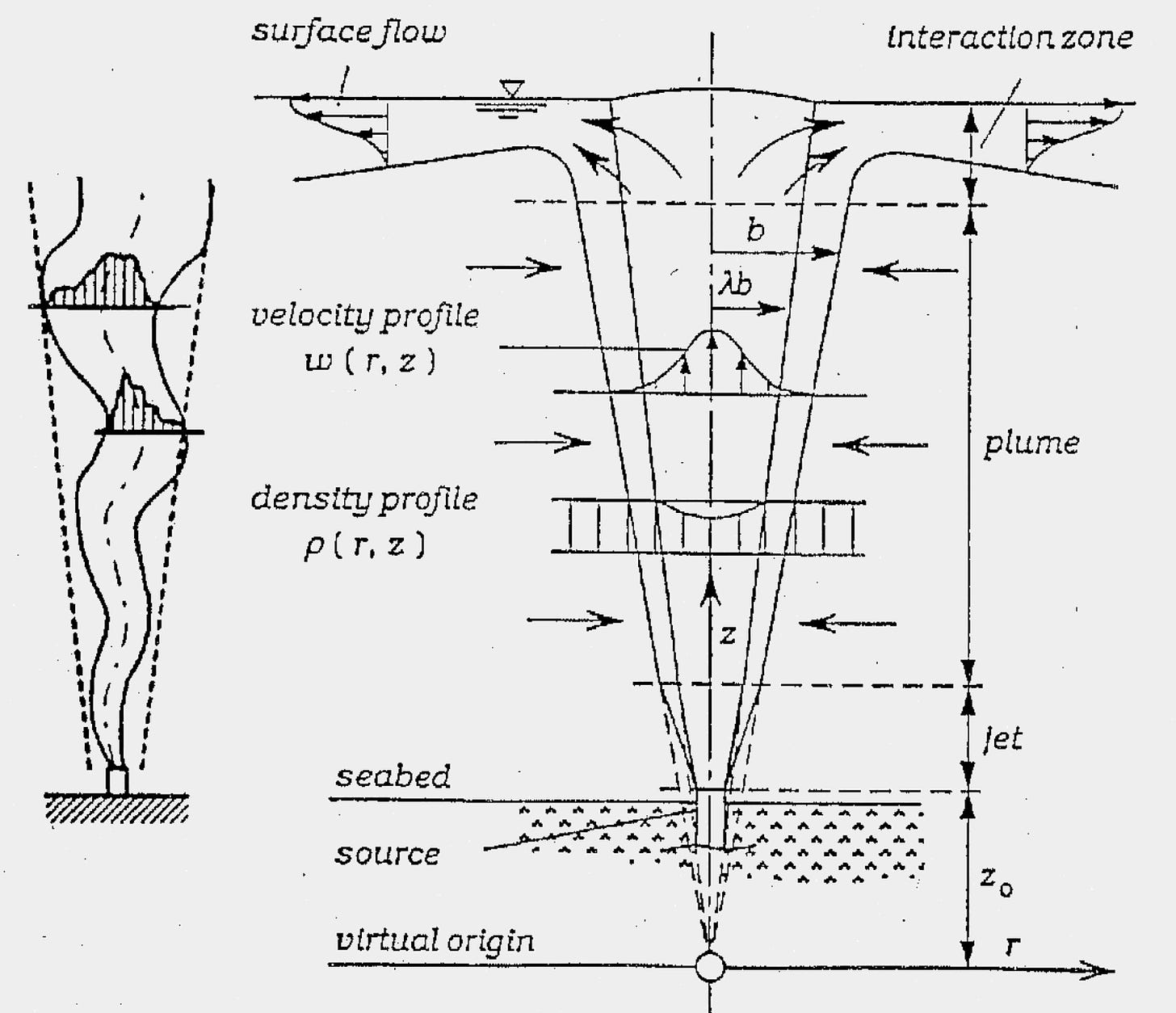
From what we can observe in the sparse footage and our flow simulations, his theory holds up well. The gas flow can be estimated from the height the fountain, but the diameter stays almost the same in a big range. Someone who didn’t get that was the Swedish coast guard who monitored the leak with fancy planes and cameras, but totally missed the only relevant metric in their public reporting.
The part I find the most interesting though is that in some of the scenarios Fanneløp and Bettelini consider, the speed of water flow is much higher at the bottom than near the surface. They also seem to think that the momentum from the gas blown out in a horizontal direction is negligible compared to the gravitational pull from the plume. The shape carved out in the hard glacial clay of the baltic seafloor supports this view.
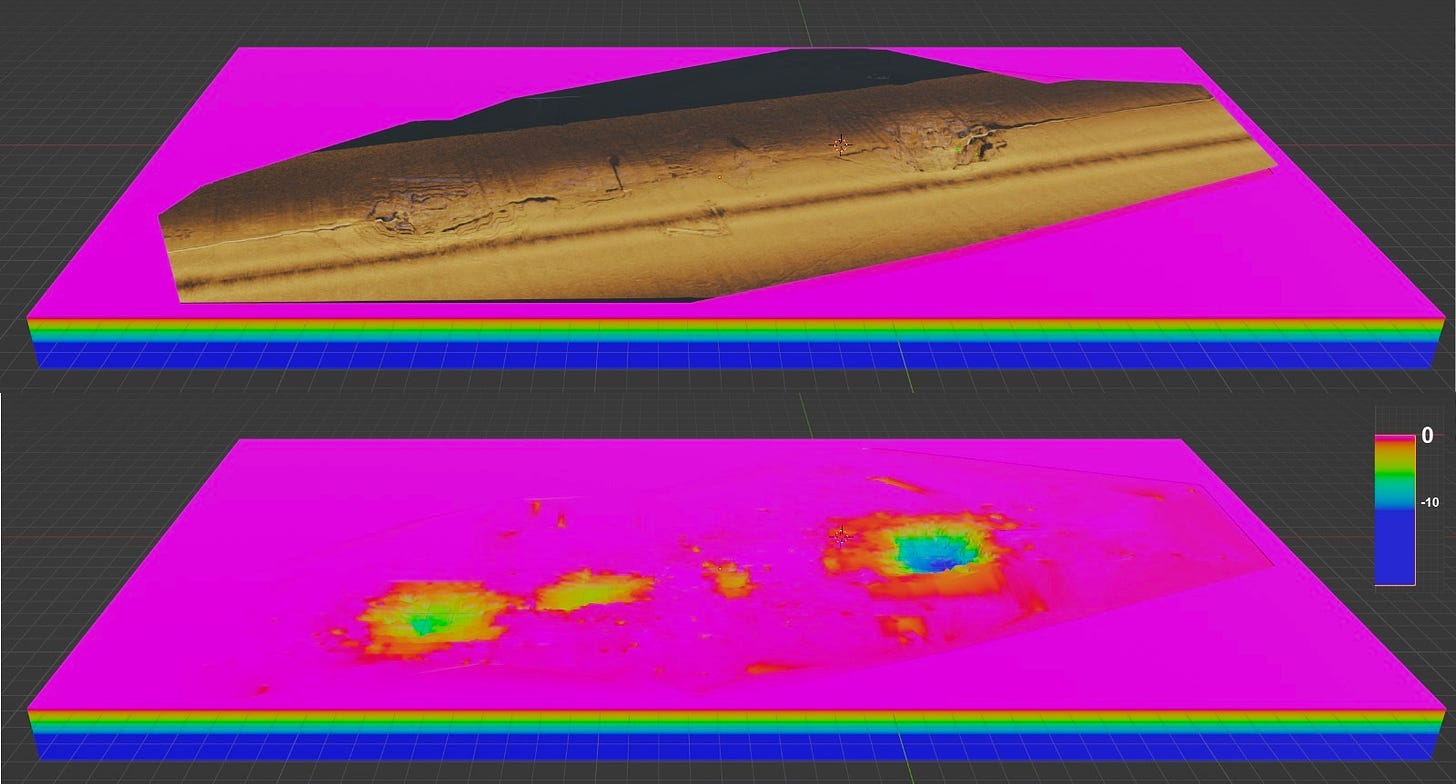
The “crater” at the Russian end is deeper but not wider than the crater around the German end of NS1S and seen above. The first half hour with many tons per second of gas blowing at sonic speed was identical between the two ends, but the Russian end continued to leak for another four days, and carved the same diameter crater twice as deep. I think maybe some of the upper soft “post glacial” mud on the seabed was blown away by the gas jet, but the carving out of the round craters in the hard glacial clay was due to the water currents driven by the buoyancy of the plume. The edges of the crater were not altered in the 4 days of additional leaking from the Russian end, and therefore I believe the water came from above, flushed the bottom and turned upwards behind the rising bubbles. The craters are round and just as steep in front as behind the flow. Therefore I believe the momentum of the water rushing in behind the bubbles dominated the momentum of the air flowing out horizontally from the pipe ends, and that happened pretty soon. I believe the bottom is shaped by fast flowing water pulled by the buoyant bubbles. It wasn’t like giant leaf-blowers blowing the mud away.
The fast moving water has another intriguing effect. Bernoullis principle tells us that the sum of kinetic energy and internal (pressure) energy stays constant in a “steady flow”. I think this is applicable here. If water flushed by the opening at speeds as high as 40 meters per second, the pressure must have been almost zero and stayed so for a long time. This made for larger bubbles and even bigger draft in the plume. The outpouring gas also stayed very cold for the first two days of leaking.
To check that the simulation was correct I requested the pressure drop curves from the Danish Energy Authority and they delivered here and here . Kudos to them. It allowed me to calibrate the pipe friction coefficient and get a good match.
A bird has told me the curves for the German side exist in a much higher resolution. I heard you can even see a spike in pressure leading the drop. Can’t wait to see that. It must be the sound of the initial chemical explosion, and it might tell us a lot we didn’t know about the bombs and the timing.
In his December 2023 Substack post on the amount of explosives used, Ola Tunander thinks the "spike" you mention at the end could have resulted from enough explosives being used to actually compress the gas. Presumably that would be a lot of explosives, giving the pipeline more of a "broadside" rather than just cutting it open. But you think of it as mere sound from the explosion?